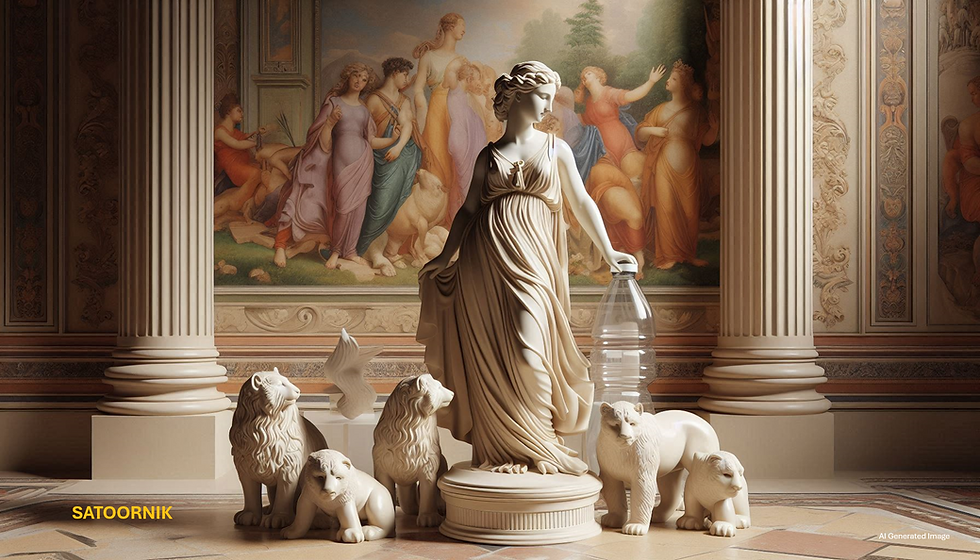
Author
Dr.-Ing. Mitra Nikpay
Published: 5.10.2024
All rights reserved © Dr.-Ing. Mitra Nikpay & SATOORNIK
1. Introduction
Microplastics (MPs) are well-known for their role as vectors of contaminants, spreading harmful chemicals across ecosystems and even into the human body. While much attention has been given to the environmental persistence of MPs, emerging research highlights four significant classes of contaminants that microplastics can carry, enhancing their impact on both the environment and public health. This article will examine how each of these groups interacts with MPs, the risks they pose, and why addressing these pollutants requires urgent attention.
2. The 4 Ps
We have identified four major pollutants of the environment: per- and polyfluoroalkyl substances (PFASs), polycyclic aromatic hydrocarbons (PAHs), pharmaceuticals, and pathogens, collectively referred to as the 4 Ps. In this section, we will explain and review how these pollutants interact with microplastics (MPs) across various environments, including water, air, and soil. Understanding these interactions is crucial for assessing the consequences of MPs as vectors for these pollutants and their broader implications for ecological and human health.
2.1. PFASs
Per- and polyfluoroalkyl substances (PFASs) are highly persistent pollutants with a broad range of industrial and consumer applications. Their environmental resilience, particularly in aquatic ecosystems, is further enhanced by their sorption onto microplastics, which act as vectors for transporting these toxic chemicals (Fig.1) [1]. Recent studies have shown that the interaction between MPs and PFAS is driven by physicochemical mechanisms, including hydrophobic interactions and partitioning. For example, polyethylene (PE) has been found to exhibit greater adsorption for PFAS than polyvinyl chloride (PVC) and polystyrene (PS), with hydrophobic PFAS showing a stronger affinity for non-polar polymers like PE [2].
The co-occurrence of MPs and PFAS in aquatic environments presents a significant risk to the environment and human health. PFAS concentrations in the air range from 0.0011 to 95.378 ng/m³, while in aquatic environments, they reach up to 268 ng/L. MPs, with concentrations as high as 4712 particles/kg in soil and 26 particles/L in water, act as persistent carriers of PFASs. Research [2] indicates that the presence of organic matter in real-world environments enhances PFAS sorption onto MPs compared to controlled conditions, potentially increasing their bioavailability and persistence in the food chain.
The toxicological risks are further increased when PFAS-laden MPs are ingested by aquatic organisms, leading to bioaccumulation and potential harm to higher trophic levels, including humans. The presence of PFAS contamination on MPs can result in prolonged environmental contamination, leading to secondary pollution and chronic exposure risks. These combined pollutants have been linked to endocrine disruption, immune suppression, and other harmful health effects in organisms [2, 3].
2.2. PAHs
Polycyclic aromatic hydrocarbons (PAHs) are a group of organic pollutants that result from the incomplete combustion of fossil fuels and have a high persistence in marine environments. It is well documented that MPs can absorb PAHs from water, creating a toxic mixture that can be easily transported across large distances in marine ecosystems. The strong chemical affinity between these contaminants is driven by hydrophobic interactions, which enable PAHs to sorb onto MPs. This allows them to be transported through the water column and taken up by marine organisms. Research has revealed that PAH concentrations on MPs in marine environments can reach as high as 120,000 ng/g, as documented in Chinese seawater. The simultaneous presence of these toxicants increases the likelihood of bioaccumulation, which presents long-term ecological and health risks [4].
The interaction between MPs and PAHs is influenced by several environmental factors, including pH, salinity, and temperature, as well as MPs' characteristics such as size, surface roughness, and aging. The presence of microbial biofilms and organic matter serves to further enhance the sorption process, thereby intensifying the persistence of these contaminants in marine ecosystems. In particular, MPs in sedimentary habitats act as vectors for PAHs, thereby increasing the risk of ingestion by non-selective feeders. This can result in bioaccumulation and oxidative stress, reproductive harm, and other toxicological effects in aquatic species [4, 5].
The combined contamination of MPs and PAHs presents a more complex remediation challenge than would be posed by their individual removal. Some studies have indicated that the biodegradation of PAHs is reduced in the presence of heavy metals. However, PAHs may enhance the photodegradation of MPs. While there are strategies for addressing PAH or MP contamination independently, there is a lack of solutions that target their mixed presence in marine systems. This gap highlights the need for new remediation techniques that can effectively address both contaminants simultaneously [4].
2.3. Pharmaceuticals
The presence of pharmaceuticals and personal care products (PPCPs) in the environment has become an increasing concern due to their potential to interact with MPs. While these compounds are typically hydrophilic, studies have shown that they can adsorb onto microplastic surfaces, facilitating their transport and persistence in aquatic ecosystems.
The sorption of PPCPs onto MPs is influenced by factors such as pH, salinity, dissolved organic matter (DOM), and the properties of both the plastics and PPCPs. While extensive research exists on hydrophobic organic contaminants (HOCs), studies focusing on hydrophilic PPCPs are limited. Recent findings suggest that polar and ionizable PPCP compounds, like antibiotics, β-blockers, antidepressants, and non-steroidal anti-inflammatory drugs (NSAIDs), interact differently with MPs depending on their charge and environmental chemistry. For instance, aged MPs, with increased surface polarity, show higher affinity for PPCPs than pristine plastics, highlighting the importance of weathering in determining sorption capacity. These interactions are critical, as they can enhance the persistence and bioavailability of PPCPs in the environment, with potential impacts on aquatic biota and human health [6].
MPs can serve as carriers of PPCPs, facilitating their movement through ecosystems and enhancing their environmental persistence. Factors such as equilibrium time, PPCP concentration, and matrix chemistry play significant roles in this vector transport. While studies have primarily focused on antibiotics, emerging research points to the need for broader investigations into other PPCP classes, such as steroidal hormones, antimicrobials, and UV-blocking agents. Moreover, the ecotoxicological effects of MP-sorbed PPCPs remain underexplored, particularly concerning their impact on human health and higher trophic levels. Research on aged MPs and environmentally relevant conditions is also sparse, highlighting the necessity for further studies on this growing issue [6, 7].
2.4. Pathogens
Microplastics not only carry chemical contaminants but also provide a substrate for the colonization of pathogens. In aquatic environments, MPs can harbor harmful microorganisms, including bacteria and viruses, increasing the potential for these pathogens to spread across ecosystems and even reach human populations. This interaction, often referred to as the "plastisphere," highlights how MPs can facilitate the long-range transmission of waterborne diseases.
Studies show that MPs act as long-term substrates, capable of hosting a diverse array of pathogenic species for extended periods, such as Vibrio bacteria, commonly found in marine environments. Viruses, like bacteria, can adhere to the surface of MPs in aquatic environments. Plastic surfaces provide a stable environment for viruses to persist longer than in free water, enhancing their survival and potential for transmission. Research has shown that certain viruses, such as influenza A and B and SARS-CoV-2, can survive for days on plastic surfaces, increasing the risk of human exposure through direct contact or ingestion of contaminated water [8, 9].
The extent to which MPs serve as vectors for pathogens remains a topic of discussion, but studies indicate that certain settings, such as wastewater treatment plants, aquaculture farms and leachate, are the best possible hotspots for MP-mediated pathogen transmission. The implications for human health are significant, particularly as these pathogens may escape conventional water treatment processes and pose an infection risk to those who encounter contaminated water [8].
3. Remediation
To effectively address the risks associated with MPs, it is essential to prioritize the removal of MPs from the environment. This is particularly crucial given the potential for MPs to facilitate the growth, survival, and transport of a range of harmful substances, including pathogens, pollutants, persistent organic chemicals, and plastic additives (the "4 Ps").
It is important to note that the pollutants themselves, such as PFASs, PAHs, and pathogens, could potentially alter the physical and chemical properties of MPs, which may impact their separation from the environment. This highlights the need for remediation technologies to address not only the extraction of MPs but also the changing nature of the MPs due to their interactions with these pollutants.
Among the advanced solutions, magnetic separation technology like SATOORNIK Gen-I is a highly efficient method for microplastic separation. This cutting-edge approach utilizes magnetic separation techniques to target and remove microplastics (MPs) from water systems with minimal energy consumption. This technology with high efficiency over 99% addresses microplastic pollution and can adapt to the varying properties of MPs caused by pollutant interactions, guaranteeing a robust and comprehensive solution to microplastic contamination.
4. Conclusion
The presence of MPs has emerged as a significant environmental challenge, with a notable impact on both ecosystems and human health. This concise overview emphasized the multifaceted impact of MPs in facilitating the proliferation and transmission of pathogens, pollutants, persistent organic chemicals, and plastic additives, collectively known as the "4 Ps."
The evidence presented highlights the urgent need for comprehensive research to fully understand the implications of MP-associated pathogens and pollutants, including PAHs and PFASs, on public health. The current lack of knowledge regarding the direct transmission pathways of these threats requires interdisciplinary studies that bridge ecotoxicology, microbiology, and epidemiology. Furthermore, the potential for MPs to harbor antibiotic resistance genes adds another layer of complexity to the health risks posed by these pollutants, emphasizing the need for effective monitoring and intervention strategies.
To address the challenges posed by microplastics and their associated risks, it is crucial to adopt innovative remediation technologies. Approaches like magnetic separation, exemplified by SATOORNIK Tech Gen I, provide efficient and sustainable solutions for removing microplastics (MPs) from aquatic environments. These technologies address not only the immediate problem of microplastic pollution but also the broader implications related to pathogen survival, pollutant accumulation, and human health risks, thereby mitigating these issues.
In conclusion, it is imperative that researchers, policymakers, and environmental managers collaborate to prioritize the development and implementation of effective strategies for MP removal and pathogen control [11]. By addressing the root causes of microplastic pollution, we can protect ecosystems, safeguard public health, and create a cleaner, healthier environment.
References
1. Nikpay, M., 2022. Wastewater fines influence the adsorption behavior of pollutants onto microplastics. Journal of Polymers and the Environment, 30(2), pp.776-783.
2. Parashar, N., Mahanty, B. and Hait, S., 2023. Microplastics as carriers of per-and polyfluoroalkyl substances (PFAS) in aquatic environment: Interactions and ecotoxicological effects. Water Emerg. Contam. Nanoplastics, 2, p.15.
3. 3. Sobhani, Zahra, Cheng Fang, Ravi Naidu, and Mallavarapu Megharaj. "Microplastics as a vector of toxic chemicals in soil: Enhanced uptake of perfluorooctane sulfonate and perfluorooctanoic acid by earthworms through sorption and reproductive toxicity." Environmental Technology & Innovation 22 (2021): 101476.
4. Ali, M., Xu, D., Yang, X. and Hu, J., 2024. Microplastics and PAHs mixed contamination: an in-depth review on the sources, co-occurrence, and fate in marine ecosystems. Water Research, p.121622.
5. Hou, G., Zhao, X., Zhao, T., Wu, X., Pu, S., Tang, Z. and Wu, F., 2023. The adsorption of PAHs on microplastics and desorption in the simulated human digestive system. Chemical Engineering Journal, 473, p.145157.
6. Atugoda, T., Vithanage, M., Wijesekara, H., Bolan, N., Sarmah, A.K., Bank, M.S., You, S. and Ok, Y.S., 2021. Interactions between microplastics, pharmaceuticals and personal care products: Implications for vector transport. Environment International, 149, p.106367.
7. Santana-Viera, S., Montesdeoca-Esponda, S., Torres-Padrón, M.E., Sosa-Ferrera, Z. and Santana-Rodríguez, J.J., 2021. An assessment of the concentration of pharmaceuticals adsorbed on microplastics. Chemosphere, 266, p.129007
8. Zhong, H., Wu, M., Sonne, C., Lam, S.S., Kwong, R.W., Jiang, Y., Zhao, X., Sun, X., Zhang, X., Li, C. and Li, Y., 2023. The hidden risk of microplastic-associated pathogens in aquatic environments. Eco-Environment & Health.
9. Enyoh, C.E., Verla, A.W. and Verla, E.N., 2020. Novel coronavirus (SARS-CoV-2) and airborne microplastics. Journal of Materials and Environment Science, 11(9), pp.1454-1461.
10. Nikpay, M., Eqtesadi, S. and Krebs, P., 2020. Influence of synthetic wastewater on entrapped air on the isotactic and atactic polypropylene microplastic surfaces. Journal of Environmental Health Science and Engineering, 18(2), pp.1569-1579.
11. Nikpay, M. and Toorchi Roodsari, S., 2024. Crafting a Scientific Framework to Mitigate Microplastic Impact on Ecosystems. Microplastics, 3(1), pp.165-183.
Comments